Learning-Dependent Interactions Between Visual Cortex and Hippocampus
The project attempts to investigate how what we know influences the way we see, with the goal to shed light on why post traumatic stress disorder patients suffer from flashbacks, unwanted revisualization of past traumatic events. We examine the cells in the hippocampus, a brain area critical for episodic memory in humans, and the visual cortex, an area critical for how we see. The cells in these two areas are monitored online while rats respond to various behavioral manipulations. The aims are to understand (1) what aspects of visual cell properties are correlated with the final representations of memory traces in the hippocampus, and (2) what aspects of the visual cell properties are actually influenced/controlled by the hippocampal memory representations.
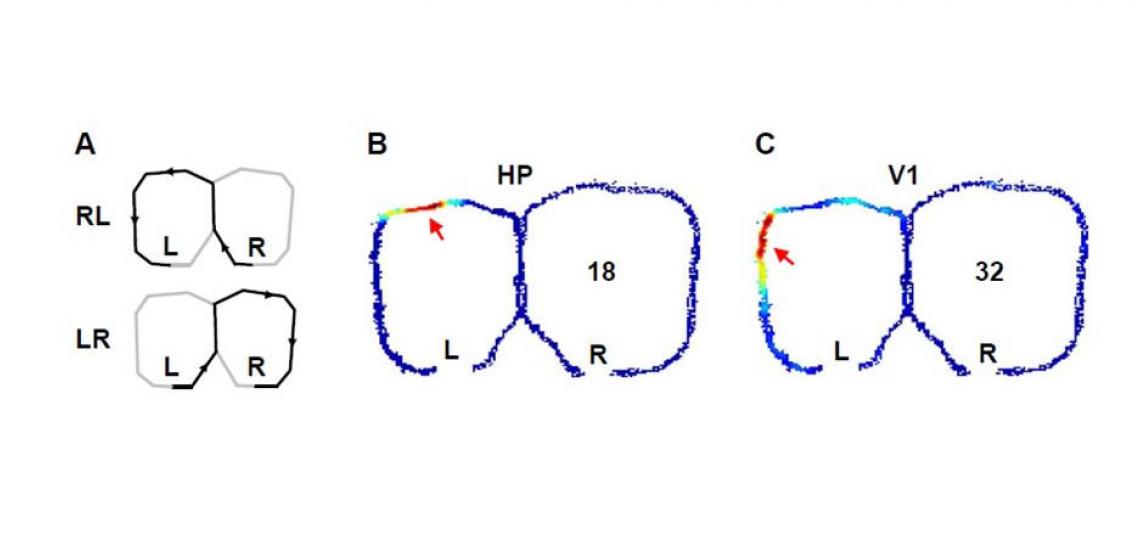
Figure 1
Figure 1: Activities of a pair of simultaneously recorded hippocampal (B) and visual cortical (C) cells while a rat was alternating two trajectories on a figure-8 shaped maze to receive food rewards at the ends (L, R) of the trajectories (A). The number of spikes per unit time fired by the two cells are color-coded. Arrows show the places on the maze where the cells fired most intensively.
We use spatial memory tasks as a model to mimic certain key aspects of the human episodic memory, such as a sequence of temporal and spatial elements in the experience. By examining the spiking activity patterns of both hippocampal and visual cortical cells (see the figure above), our plan is to uncover the correlated changes in the two areas that accompany the spatial learning.
Differential Roles of Slow-Wave Sleep and Rapid-Eye-Movement Sleep in Memory Processing
Memory benefits from a good night's sleep. How it is so remains an enigma. The memory reactivation theory proposes that one possible function of sleep is to reactivate memory traces in the hippocampus and send them back to the cortex for long-term storage. Our previous work has shown that spiking patterns representing rats’ running trajectories are indeed replayed during slow-wave sleep in both the hippocampus and visual cortex and the replays in the two areas tend to reactivate the same experience (trajectory) at the same time (see the figure below). This provides first experimental data to support the standard memory consolidation theory, which requires offline communications between the hippocampus and neocortex.
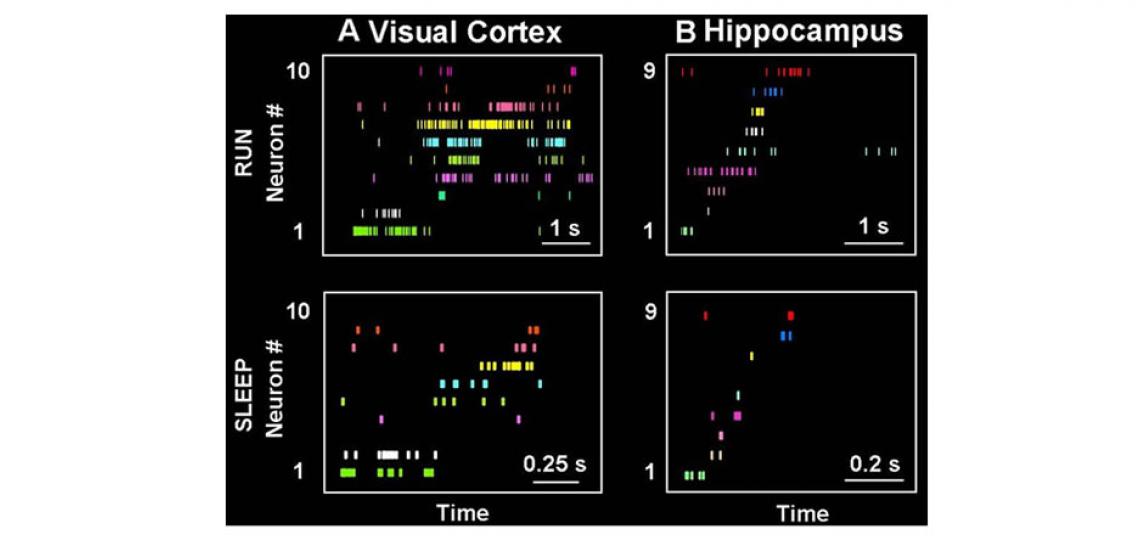
Figure 2
Figure 2: Memory traces are replayed during sleep. (A, B) Activity patterns in the visual cortex (A) and hippocampus (B) during run and sleep. A particular experience, such as running a trajectory on a maze, is encoded by unique spike patterns (RUN) of populations of neurons in both areas. Each row represents a neuron and each tick represents a spike at a particular time of the running. Later during slow-wave sleep, similar activity patterns are re-expressed (SLEEP) in both the visual cortex and hippocampus.
An interesting question is why we have two major sleep stages, rapid-eye movement sleep, when long, event-like dreams occur and non-rapid-eye movement sleep (a major component is slow-wave sleep) when only fragmented, short dream episodes, if any, occur. Both sleep stages are beneficial to memory, yet they are very different in terms of overall brain activity and perceptual correlates. This project intends to study the differences between SWS and REM in (1) the way the visual cortex and hippocampus communicate, and (2) the properties of synaptic plasticity that may be involved in sleep information processing.
Abnormal Spatial Memory Processing in Neurological and Psychiatric Disorders
Abnormal memory formation or recall is a major aspect of a number of neurological and psychiatric disorders, including Alzheimer’s disease, post traumatic syndrome disorder, and intellectual disability disorders. Rapid advances have been made in understanding their pathological causes. However, how the behavioral symptoms are produced in the living brain with ongoing pathogenesis has not been studied in most of these disorders. We utilize available rodent models of these disorders, transgenic or pharmacological, and aim to understand how the disease pathogenesis correlates with the functional changes in the memory circuits of live, behaving animals, which are ultimately responsible for producing the behavioral deficits.
For example, our recent work on a mouse model of tau-mediated neurodegeneration, a key pathology of Alzheimer’s disease, reveals a surprising functional change of hippocampal neurons. In normal, healthy animals, hippocampal neurons encode a specific experience with a precise, unique spiking pattern. However, the neurons in the tau mouse model form “rigid” spike patterns that fail to distinguish different experiences (Figure 3). The result suggests that the tau pathology makes the memory circuits stuck with existing memories and thus unable to form new memories.
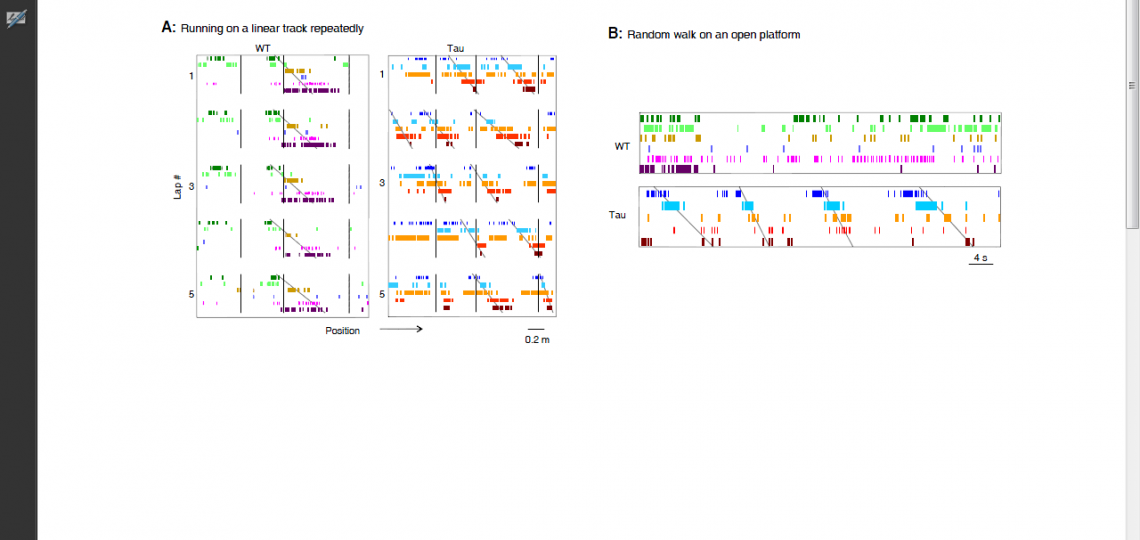
Figure 3
Figure 3: Rigid spiking patterns of hippocampal neurons in a mouse model of tau-mediated neurodegeneration. (A) Spiking patterns of hippocampal neurons in a wildtype mouse (WT) and in a mouse with tau neurodegeneration (Tau), when the animals repeatedly run on a linear track lap by lap. Each row represents a neuron and each tick represents a spike at a particular position of the track. Vertical lines mark the landmarks on the track. The WT neurons fire one after another with a sequence (marked by an angled line), which is locked to a fixed portion of the track during every lap of the running (5 laps are shown). The Tau neurons still form a sequence, but shift their spiking locations lap by lap. (B) Spiking patterns of the same WT and Tau hippocampal neurons when the animals later freely explore an open platform. The same sequence of the Tau neurons, but not that of the WT neurons, occurs on the open platform. Therefore, unlike WT ones, the Tau neurons form rigid firing patterns that do not distinguish different parts of the linear track or two very different environments.
Figure 3: Rigid spiking patterns of hippocampal neurons in a mouse model of tau-mediated neurodegeneration. (A) Spiking patterns of hippocampal neurons in a wildtype mouse (WT) and in a mouse with tau neurodegeneration (Tau), when the animals repeatedly run on a linear track lap by lap. Each row represents a neuron and each tick represents a spike at a particular position of the track. Vertical lines mark the landmarks on the track. The WT neurons fire one after another with a sequence (marked by an angled line), which is locked to a fixed portion of the track during every lap of the running (5 laps are shown). The Tau neurons still form a sequence, but shift their spiking locations lap by lap. (B) Spiking patterns of the same WT and Tau hippocampal neurons when the animals later freely explore an open platform. The same sequence of the Tau neurons, but not that of the WT neurons, occurs on the open platform. Therefore, unlike WT ones, the Tau neurons form rigid firing patterns that do not distinguish different parts of the linear track or two very different environments.