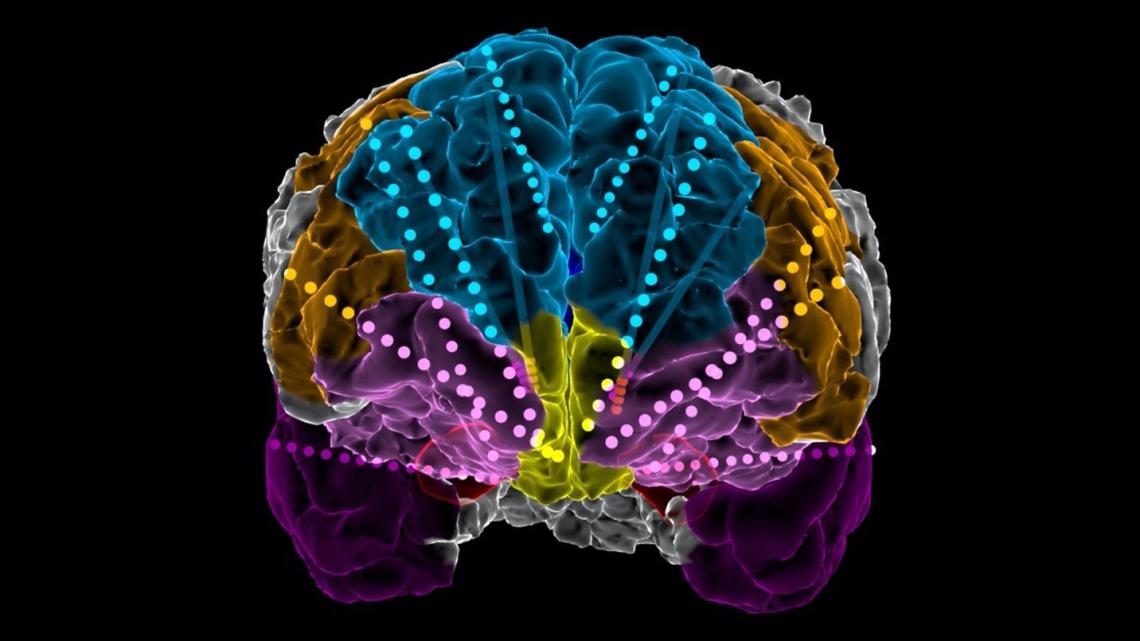
Investigating DBS for Treatment-Resistant Depression
A New Platform Based on Individualized Intracranial Recording and Stimulation
The success of deep brain stimulation (DBS) for movement disorders has encouraged many groups around the world to apply the DBS platform to treat severe and refractory psychiatric disorders. We are running an NIH BRAIN Initiative funded (UH3 NS103549) trial of DBS for treatment-resistant depression (TRD). This trial focuses on depression patients who have tried and failed conventional treatments, including anti-depressant medications, behavioral therapy, and electroconvulsive therapy. Sameer Sheth is the contact principle investigator and co-primary investigators are Wayne Goodman, M.D., professor and chair of psychiatry at Baylor College of Medicine, and Nader Pouratian, M.D., Ph.D., professor and chair of neurological surgery at UT Southwestern Medical Center
Early open-label trials of DBS for TRD demonstrated promising results, but two large industry-sponsored pivotal trials were halted at interim analyses and were not able to substantiate efficacy. With this history as context, we planned our current trial with a different approach in mind. Patients with the same diagnosis of TRD may yet have very different constellations of symptoms, different “phenotypes.” We reason that these different phenotypes are manifestations of different brain network activity patterns across individuals. Thus, to optimize delivery of DBS therapy to a particular individual, we have to derive a detailed understand of his/her depression-relevant brain networks, such as those regulating mood, affect, anxiety, attention, cognition, etc. The better we understand these pathological networks within the individual, the better we will be able to engage them and restore balanced, healthy function.
To this end, we borrow an approach common to epilepsy surgery, but not previously applied to most other neurological or psychiatric conditions. We employ semi-chronically implanted stereo-EEG (sEEG) electrodes to perform intracranial recordings and stimulation to understand network activity at the individual patient level. TRD patients in this trial are implanted with DBS electrodes targeting two previously studied targets, the ventral capsule/ventral striatum (VCVS) and subgenual cingulate (SGC). We also temporarily implant sEEG electrodes in depression-relevant regions (prefrontal, mesial temporal, etc.) and keep the patient in the epilepsy monitoring unit (EMU) for 1.5 weeks. During this time, we perform a number of recording and stimulation studies to understand the patient’s network activity and how it responds to stimulation. Patients then transition to the outpatient phase, during which we use the EMU-derived information to optimize programming of the DBS system. We expect that this trial will provide an unparalleled understanding of symptomatic networks in TRD and how they can be engaged and manipulated back to a healthy state with individualized DBS. More broadly, we hope that this approach of using the intracranial EEG platform can be applied to many other neurological and psychiatric disorders whose circuit-level dysfunction remains incompletely understood.
For more information: View the clinical trials listing.
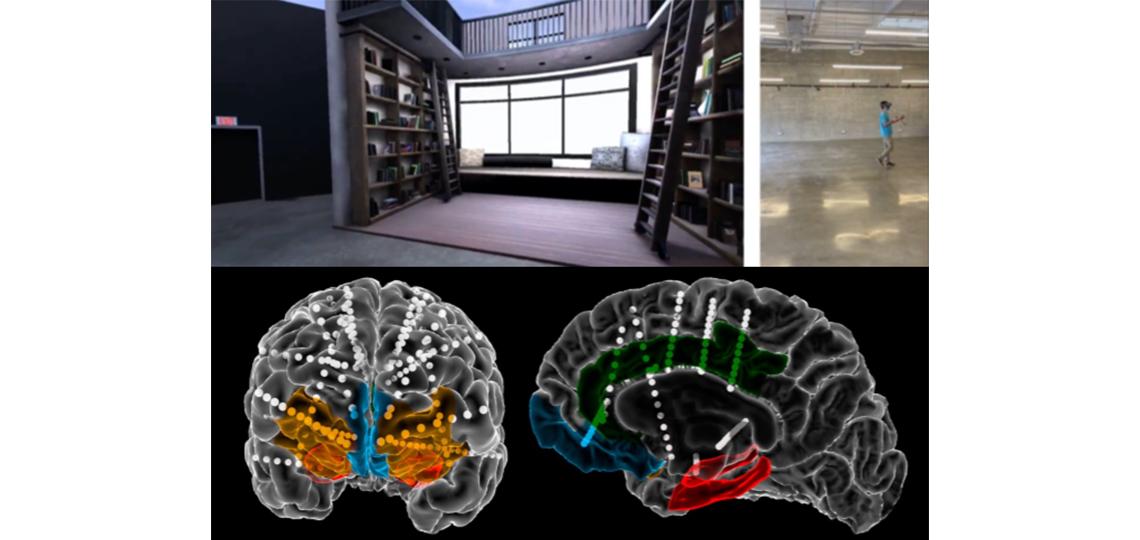
Investigating the Neural Mechanisms Underlying Ad-hoc Self-Programmability
Human beings possess the remarkable ability to hear a series of instructions for the first time and implement them without practice and with a high degree of accuracy. How do our brains afford us this tremendous flexibility? This ability to ‘re-program’ ourselves has so far eluded artificial intelligence systems, and its underlying neural implementation in biological brains remains unknown.
We aim to explore this question using a novel behavioral task, neurosurgical recordings of neural activity, and advanced neural network modeling. Patients are asked to perform a complex rule-following task where the rule changes dynamically from one trial to the next, and each rule contains more than one sub-rule that determines the mapping between incoming stimuli and participants’ responses. While patients perform this task, we record neural activity from medio-temporal and prefrontal regions putatively involved in higher-level reasoning and procedural learning.
We are interested in whether individual neurons in these regions keep track of where we are in ‘cognitive’ space. We are also interested in whether some neurons represent rule structures more generally, independent of the specific stimuli that instantiate them. How do these individual neurons then form circuits that can dynamically interpret sensory stimuli in light of the ongoing rule, and dynamically reconfigure their activity to represent new information? How does the network switch back and forth between different sub-rules without interference? What neural signatures are associated with errors that would indicate a failure of this process?
In collaboration with researchers at Google DeepMind, Columbia University, UCLA, University of Utah and Oxford University we hope to further understand this process by simulating it in silico using deep learning models. Recent artificial neural networks models of brain circuits have shed light on the circuit mechanisms underlying a myriad of perceptual and cognitive processes - all the way from basic processing in the retina to complex, context-dependent perceptual decision-making. Using the same models that have led to remarkable advances in AI - deep reinforcement learning models - we hope to better understand the brain mechanisms underlying this remarkable human ability.
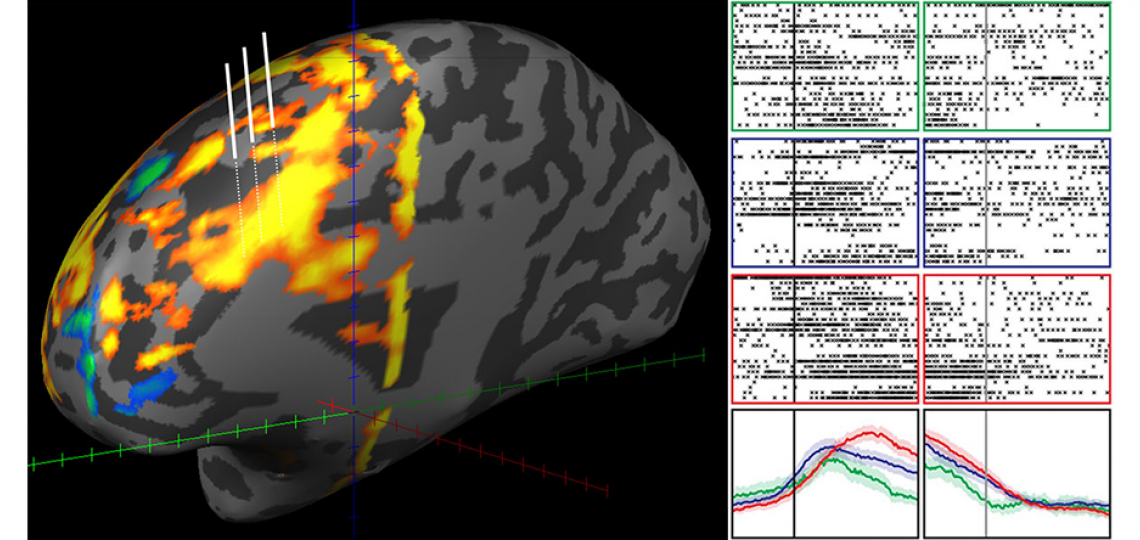
Investigating the Physiology of Controlled Decision-Making in Human Prefrontal Cortex
Every day we are faced with decisions that require fast but accurate actions. ‘Cognitive control’ refers to our ability to optimize our behavior to meet the demands of our ever-changing world. For example, when driving in traffic, we must pay attention to relevant factors such as speed, distance to destination and the presence of other drivers. We must also prevent ourselves from being too distracted by signals that are irrelevant to driving, such as music or children arguing with each other in the back seat. All of these dynamic processes occur while keeping our long-term goals in mind. The human prefrontal cortex (PFC) is the most evolutionarily advanced region of our brains. Across thousands of years, the PFC has developed specialized neuronal networks for making quick and accurate decisions. The PFC also coordinates with brain regions such as the hippocampus to encode spatial and memory representations. In our lab, we have designed complex cognitive tasks that evoke distinct components of cognitive control and decision-making. These tasks have rules that change after a certain number of iterations. We seek to answer questions such as, 'How does the brain quickly adjust to new scenarios and stimulus-response patterns?' We use a combination of single-unit and local field potential (LFP) recordings from neurosurgical patients to answer these questions.
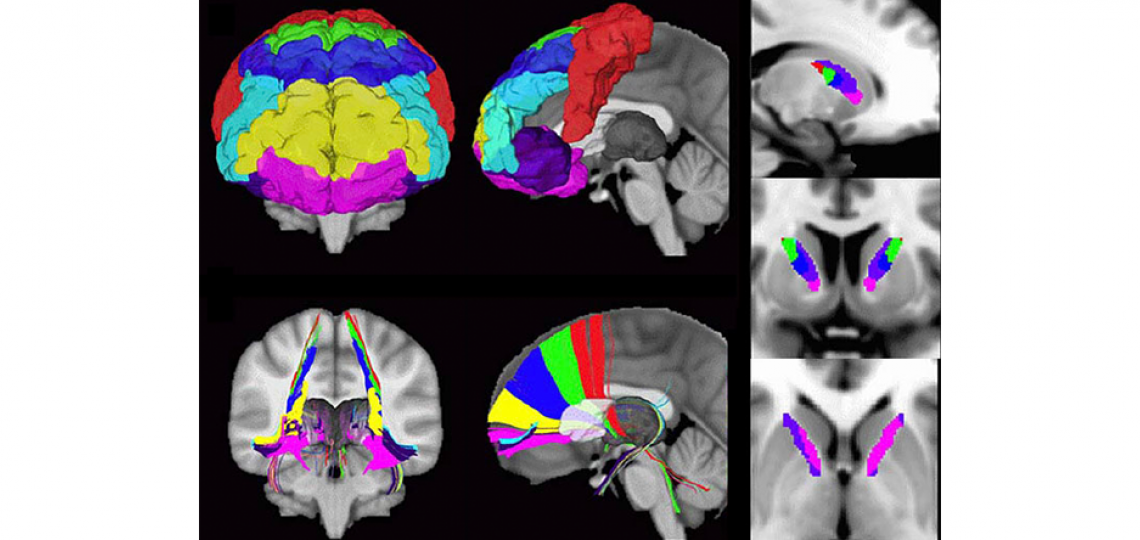
Investigating Cortical and Basal Ganglia Networks Involved in Disorders of Mental Health
Patients with certain psychiatric disorders that are resistant to conventional treatment strategies may be candidates for surgical intervention. Studies over the past decade have shown extremely promising results for the surgical treatment of obsessive-compulsive disorder (OCD) and major depressive disorder (MDD), and many others are under investigation. The two types of surgical procedures currently available are stereotactic lesions and deep brain stimulation (DBS). By their stereotactic nature, both of these procedures target specific brain regions, putative nodes in a behavioral circuit whose function has gone awry. We use imaging analysis techniques such as diffusion tensor imaging (DTI), task-based and resting state functional MRI to study the structure of cortical and subcortical circuits in individuals with OCD, MDD, and other psychiatric conditions. We are interested in identifying structural or functional predictors of clinical response, as well as studying longitudinal changes over time.
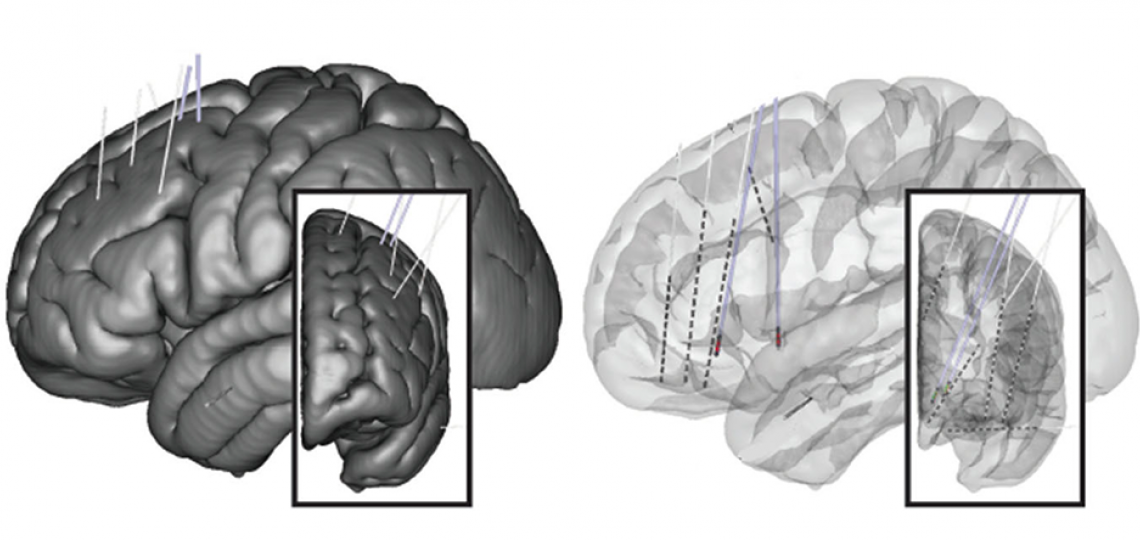
Developing Novel Targets and Indications for Neuromodulation
The field of neuromodulation is undergoing a rapid expansion. Experimental studies and clinical trials are underway for a variety of neurological and psychiatric diseases. We collaborate closely with clinicians and scientists in the Departments of Psychiatry, Neurology, Neuroscience, Engineering, and others to develop new targets and indications for neuromodulation. Parallel investigations across species models and scale (synapses, cells, networks, behavior) allow information to flow back-and-forth between the lab and clinic, facilitating the advancement of our understanding of the system and how to therapeutically modulate it.
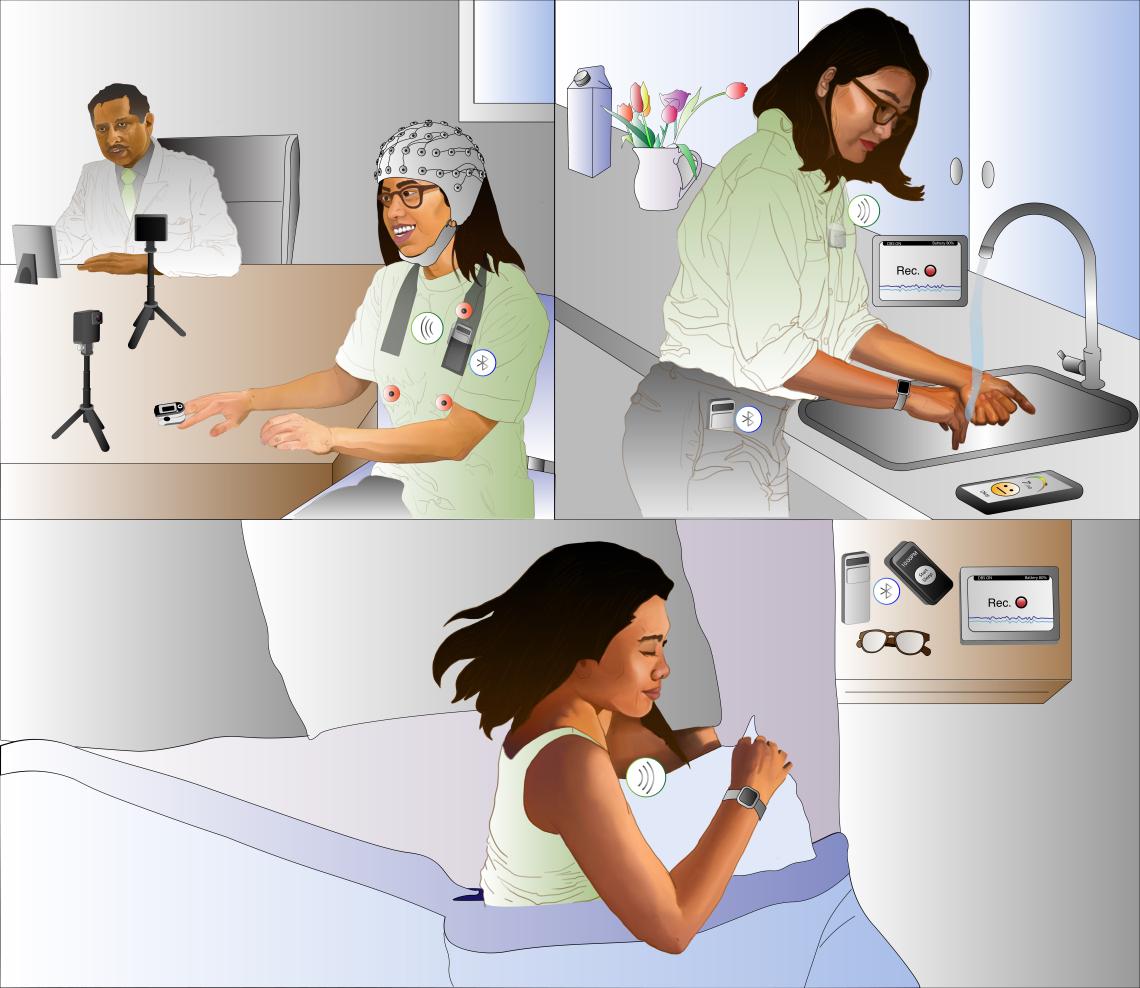
Developing Adaptive Deep Brain Stimulation for Treatment-Resistant Obsessive-Compulsive Disorder
Deep brain stimulation (DBS) of the ventral capsule/ventral striatum (VC/VS) improves symptoms in ~66% of patients with treatment-resistant obsessive-compulsive disorder (OCD). Conventional stimulation paradigms for OCD treatment are open-loop, continuous DBS (cDBS). Stimulation parameter adjustments require the patient to visit their psychiatrist in the clinic. Programming visits are burdensome and time intensive for both patients and clinicians. Our study aims (Grant: UH3NS100549) to develop closed-loop, adaptive DBS (aDBS), in which stimulation would be automatically titrated in response to specific symptom/side effect states. An aDBS system would decrease programming burden and increase efficacy of DBS for OCD.
Neural biomarkers of OCD symptom severity and side effects of VC/VS must be identified in OCD to develop aDBS algorithms that improve on the traditional cDBS paradigm. The development of bidirectional DBS devices has equipped us to stream neural data chronically while delivering therapeutic stimulation. We collect these data in the clinic and at home in naturalistic settings. Integrating in-clinic and at-home data will inform our understanding of patient-specific pathology and allow us to investigate how OCD affects brain networks across patients.